Details:Physics: Unterschied zwischen den Versionen
Tes (Diskussion | Beiträge) |
Tes (Diskussion | Beiträge) |
||
Zeile 26: | Zeile 26: | ||
====2.1 Heat Losses due to moisture==== | ====2.1 Heat Losses due to moisture==== | ||
[[Bild:PhysicalBackgroundFig1.jpg| | [[Bild:PhysicalBackgroundFig1.jpg|thumb|left]]The heat and moisture transport processes in buildings are usually strongly '''coupled'''. This is particularly evident in the effect of moisture on the heat insulation of building components. Fig. 1 shows the increase in heat conductivity of three different building materials as dependent on their moisture content [1]. | ||
The heat and moisture transport processes in buildings are usually strongly '''coupled'''. This is particularly evident in the effect of moisture on the heat insulation of building components. Fig. 1 shows the increase in heat conductivity of three different building materials as dependent on their moisture content [1]. | |||
While the heat conductivity of mineral materials, such as the cellular concrete shown here, increases linearly with moisture content, the heat conductivity of polystyrene foam shows a slightly progressive increase. Surprisingly, it takes only a very low moisture content to increase the heat conductivity of mineral wool markedly. This is due to the pronounced moisture redistribution by vapor diffusion in the mineral wool when a temperature gradient is applied across the sample. These are so-called non-steady latent heat effects, due to phase changes of the moisture in the material during the measurement in the guarded hot plate apparatus. These latent heat effects are usually of short duration only and have nothing to do with the true heat conductivity of the insulation material. Since their effect depends strongly on the materials adjacent to the insulation layer, they are not a characteristic of the insulation material itself [2]. Therefore the heat conductivity of mineral wool determined in this way and shown in Fig. 1 is not well suited as a material property function for non-steady calculations or for determining the steady-state U-factor. The true heat conductivity of mineral wool can be determined in the guarded hot plate apparatus if appropriate precautions are employed. Results of such measurements demonstrate that if latent heat effects are excluded, the heat conductivity of mineral wool shows a moisture dependence very similar to that of the polystyrene foam in Fig. 1. Similar remarks apply to other permeable insulation materials. | While the heat conductivity of mineral materials, such as the cellular concrete shown here, increases linearly with moisture content, the heat conductivity of polystyrene foam shows a slightly progressive increase. Surprisingly, it takes only a very low moisture content to increase the heat conductivity of mineral wool markedly. This is due to the pronounced moisture redistribution by vapor diffusion in the mineral wool when a temperature gradient is applied across the sample. These are so-called non-steady latent heat effects, due to phase changes of the moisture in the material during the measurement in the guarded hot plate apparatus. These latent heat effects are usually of short duration only and have nothing to do with the true heat conductivity of the insulation material. Since their effect depends strongly on the materials adjacent to the insulation layer, they are not a characteristic of the insulation material itself [2]. Therefore the heat conductivity of mineral wool determined in this way and shown in Fig. 1 is not well suited as a material property function for non-steady calculations or for determining the steady-state U-factor. The true heat conductivity of mineral wool can be determined in the guarded hot plate apparatus if appropriate precautions are employed. Results of such measurements demonstrate that if latent heat effects are excluded, the heat conductivity of mineral wool shows a moisture dependence very similar to that of the polystyrene foam in Fig. 1. Similar remarks apply to other permeable insulation materials. |
Version vom 6. Juli 2009, 13:47 Uhr
Moisture Transport in Building Materials
Computer Simulation with the WUFI Model
1. Introduction
For the practitioner the subject "moisture transport in building materials" mainly evokes vapor diffusion, dew point and the Glaser method described in German standard DIN 4108. Once a building element has been classified as "safe according to Glaser", all is over and done with as far as the designer is concerned.
Only if unexpected moisture damage occurs nevertheless, or if the designed building element does not pass the standard Glaser assessment, the search for alternative assessment methods begins. Since condensation by vapor transport in winter (which is what Glaser investigates) is only one of a large number of possible moisture loads on a building element, a positive assessment according to DIN 4108 may imply moisture safety which does not really exist. Possible problems with other hygric processes, such as indoor air convection, precipitation or rising damp, are usually not considered. The same goes for construction moisture which, in view of today's deadline pressure, causes an increasing number of damage cases. In order to allow for these effects too, one has to pass on from Glaser's simple steady-state assessment method to the realistic simulation of hygric processes in building elements. To this end, new non-steady simulation methods have been developed and experimentally validated in recent years, whose reliability is winning them more and more acceptance among practitioners. This fact is also recognized in the new draft for DIN 4108-3 which now admits these methods.
In the following we will demonstrate the effects of increased moisture levels and of alternating hygric stresses, and we will describe the basic physics of hygric processes in building elements. Subsequently, we will analyse the necessary climate and material data and discuss the accuracy of the calculation, using the non-steady simulation model WUFI as an example which has meanwhile found widespread use.
2. Hygric Effects in Building Components
The fitness for use and the durability of building components and building materials may be affected by moisture, as shown by these examples:
- reduction of thermal insulation
- increased dust contamination, algae or mold growth
- mechanical stresses through swelling and shrinking caused by changes in humidity or by salt crystallization
- damages due to frost, rotting or corrosion, caused by increased moisture in the material
- incomplete hydratation because of drying too rapidly
- delayed maturing of screed topping because of drying too slowly
2.1 Heat Losses due to moisture
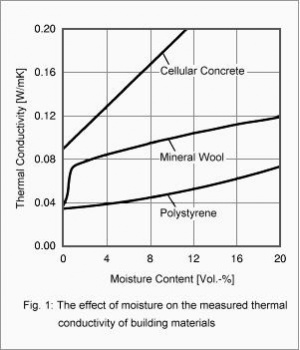
The heat and moisture transport processes in buildings are usually strongly coupled. This is particularly evident in the effect of moisture on the heat insulation of building components. Fig. 1 shows the increase in heat conductivity of three different building materials as dependent on their moisture content [1].
While the heat conductivity of mineral materials, such as the cellular concrete shown here, increases linearly with moisture content, the heat conductivity of polystyrene foam shows a slightly progressive increase. Surprisingly, it takes only a very low moisture content to increase the heat conductivity of mineral wool markedly. This is due to the pronounced moisture redistribution by vapor diffusion in the mineral wool when a temperature gradient is applied across the sample. These are so-called non-steady latent heat effects, due to phase changes of the moisture in the material during the measurement in the guarded hot plate apparatus. These latent heat effects are usually of short duration only and have nothing to do with the true heat conductivity of the insulation material. Since their effect depends strongly on the materials adjacent to the insulation layer, they are not a characteristic of the insulation material itself [2]. Therefore the heat conductivity of mineral wool determined in this way and shown in Fig. 1 is not well suited as a material property function for non-steady calculations or for determining the steady-state U-factor. The true heat conductivity of mineral wool can be determined in the guarded hot plate apparatus if appropriate precautions are employed. Results of such measurements demonstrate that if latent heat effects are excluded, the heat conductivity of mineral wool shows a moisture dependence very similar to that of the polystyrene foam in Fig. 1. Similar remarks apply to other permeable insulation materials.